A tissue sample and a biodegradable scaffold were used to grow vaginas in the right size and shape for each woman as well as being a tissue match. Experts said the study, published in the Lancet, was the latest example of the power of regenerative medicine
'I feel fortunate'
In each woman the vagina did not form properly while they were still inside their mother's womb, a condition known as vaginal aplasia. Current treatments can involve surgically creating a cavity, which is then lined with skin grafts or parts of the intestine. Doctors at Wake Forest Baptist Medical Centre in North Carolina used pioneering technology to build vaginas for the four women who were all in their teenage years at the time. Scans of the pelvic region were used to design a tube-like 3D-scaffold for each patient. A small tissue biopsy was taken from the poorly developed vulva and grown to create a large batch of cells in the lab. Muscle cells were attached to the outside of the scaffold and vaginal-lining cells to the inside. The vaginas were carefully grown in a bioreactor until they were suitable to be surgically implanted into the patients. One of the women with an implanted vagina, who wished to keep her name anonymous, said: "I believe in the beginning when you find out you feel different. "I mean while you are living the process, you are seeing the possibilities you have and all the changes you'll go through. "Truly I feel very fortunate because I have a normal life, completely normal."
'An important thing'
All the women reported normal sexual function. Vaginal aplasia can lead to other abnormalities in the reproductive organs, but in two of the women the vagina was connected to the uterus. There have been no pregnancies, but for those women it is theoretically possible.
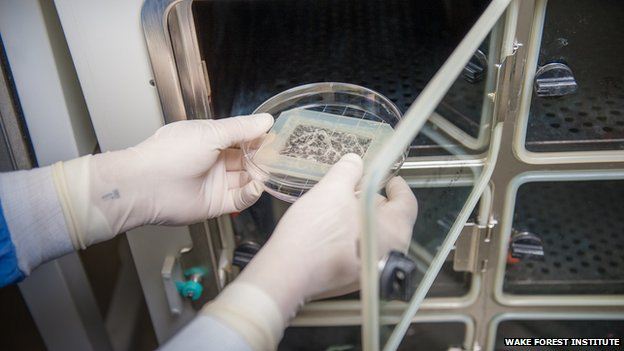
Dr Anthony Atala, director of the Institute for Regenerative Medicine at Wake Forest, told the BBC News website: "Really for the first time we've created a whole organ that was never there to start with, it was a challenge." He said a functioning vagina was a "very important thing" for these women's lives and witnessing the difference it made to them "was very rewarding to see". This is the first time the results have been reported. However, the first implants took place eight years ago.
'Most important questions'
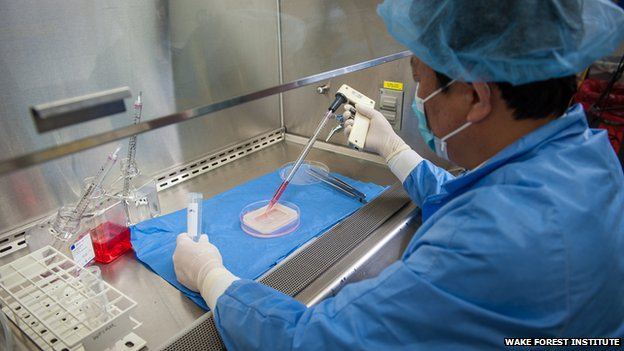
Meanwhile, researchers at the University of Basel in Switzerland have used similar techniques to reconstruct the noses of patients after skin cancer. It could replace the need to take cartilage from the ribs or ears in order to rebuild the damage caused by cutting the cancer away. Prof Martin Birchall, who has worked on lab-grown windpipes, commented: "These authors have not only successfully treated several patients with a difficult clinical problem, but addressed some of the most important questions facing translation of tissue engineering technologies. The steps between first-in-human experiences such as those reported here and their use in routine clinical care remain many, including larger trials with long-term follow-up, the development of clinical grade processing, scale-out, and commercialisation."
sci-hub.org/downloads/1d30/raya-rivera2014.pdf
Human lungs in the lab. For the first time, scientists have created human lungs in a lab — an exciting step forward in regenerative medicine, but an advance that likely won’t help patients for many years.
“It’s so darn cool,” said Joan Nichols, a researcher at the University of Texas Medical Branch. “It’s been science fiction and we’re moving into science fact.”
If the lungs work — and that’s a big if — they could help the more than 1,600 people awaiting a lung transplant. Lungs are one of many body parts being manufactured in the lab — some parts, such as tracheas and livers, are even further along.
“Whole-organ engineering is going to work as a solution to the organ donor shortage,” said Dr. Stephen Badylak, deputy director of the McGowan Institute for Regenerative Medicine at the University of Pittsburgh.
The researchers in Galveston, Texas, started with lungs from two children who’d died from trauma, most likely a car accident, Nichols said. Their lungs were too damaged to be used for transplantation, but they did have some healthy tissue.
They took one of the lungs and stripped away nearly everything, leaving a scaffolding of collagen and elastin.
The scientists then took cells from the other lung and put them on the scaffolding. They immersed the structure in a large chamber filled with a liquid “resembling Kool-Aid,” Nichols said, which provided nutrients for the cells to grow. After about four weeks, an engineered human lung emerged.
Repeating the process, they created another lung from two other children who’d died.
The lab-made lungs look very much like the real thing, Nichols says, just pinker, softer and less dense.
Nichols said she thinks it will be another 12 years or so until they’ll be ready to try using these lungs for transplants.
“My students will be doing the work when I’m old and retired and can’t hold a pipette anymore,” she said.
Before researchers experiment on humans, they’ll try out lab-made lungs on pigs, she said.
Picture UTMB: The lab-grown lung before and after being reseeded with cells. Image
The 2013 Nobel Prize in Physiology or Medicine: James E. Rothman, Randy W. Schekman and Thomas C. Südhof for their discoveries of machinery regulating vesicle traffic, a major transport system in our cells
The 2013 Nobel Prize honours three scientists who have solved the mystery of how the cell organizes its transport system. Each cell is a factory that produces and exports molecules. For instance, insulin is manufactured and released into the blood and chemical signals called neurotransmitters are sent from one nerve cell to another. These molecules are transported around the cell in small packages called vesicles. The three Nobel Laureates have discovered the molecular principles that govern how this cargo is delivered to the right place at the right time in the cell.
Randy Schekman discovered a set of genes that were required for vesicle traffic. James Rothman unravelled protein machinery that allows vesicles to fuse with their targets to permit transfer of cargo. Thomas Südhof revealed how signals instruct vesicles to release their cargo with precision.
Through their discoveries, Rothman, Schekman and Südhof have revealed the exquisitely precise control system for the transport and delivery of cellular cargo. Disturbances in this system have deleterious effects and contribute to conditions such as neurological diseases, diabetes, and immunological disorders.
How cargo is transported in the cell
In a large and busy port, systems are required to ensure that the correct cargo is shipped to the correct destination at the right time. The cell, with its different compartments called organelles, faces a similar problem: cells produce molecules such as hormones, neurotransmitters, cytokines and enzymes that have to be delivered to other places inside the cell, or exported out of the cell, at exactly the right moment. Timing and location are everything. Miniature bubble-like vesicles, surrounded by membranes, shuttle the cargo between organelles or fuse with the outer membrane of the cell and release their cargo to the outside. This is of major importance, as it triggers nerve activation in the case of transmitter substances, or controls metabolism in the case of hormones. How do these vesicles know where and when to deliver their cargo?
Traffic congestion reveals genetic controllers
Randy Schekman was fascinated by how the cell organizes its transport system and in the 1970s decided to study its genetic basis by using yeast as a model system. In a genetic screen, he identified yeast cells with defective transport machinery, giving rise to a situation resembling a poorly planned public transport system. Vesicles piled up in certain parts of the cell. He found that the cause of this congestion was genetic and went on to identify the mutated genes. Schekman identified three classes of genes that control different facets of the cell´s transport system, thereby providing new insights into the tightly regulated machinery that mediates vesicle transport in the cell.
Docking with precision
James Rothman was also intrigued by the nature of the cell´s transport system. When studying vesicle transport in mammalian cells in the 1980s and 1990s, Rothman discovered that a protein complex enables vesicles to dock and fuse with their target membranes. In the fusion process, proteins on the vesicles and target membranes bind to each other like the two sides of a zipper. The fact that there are many such proteins and that they bind only in specific combinations ensures that cargo is delivered to a precise location. The same principle operates inside the cell and when a vesicle binds to the cell´s outer membrane to release its contents.
It turned out that some of the genes Schekman had discovered in yeast coded for proteins corresponding to those Rothman identified in mammals, revealing an ancient evolutionary origin of the transport system. Collectively, they mapped critical components of the cell´s transport machinery.
Timing is everything
Thomas Südhof was interested in how nerve cells communicate with one another in the brain. The signalling molecules, neurotransmitters, are released from vesicles that fuse with the outer membrane of nerve cells by using the machinery discovered by Rothman and Schekman. But these vesicles are only allowed to release their contents when the nerve cell signals to its neighbours. How is this release controlled in such a precise manner? Calcium ions were known to be involved in this process and in the 1990s, Südhof searched for calcium sensitive proteins in nerve cells. He identified molecular machinery that responds to an influx of calcium ions and directs neighbour proteins rapidly to bind vesicles to the outer membrane of the nerve cell. The zipper opens up and signal substances are released. Südhof´s discovery explained how temporal precision is achieved and how vesicles´ contents can be released on command.
Vesicle transport gives insight into disease processes
The three Nobel Laureates have discovered a fundamental process in cell physiology. These discoveries have had a major impact on our understanding of how cargo is delivered with timing and precision within and outside the cell. Vesicle transport and fusion operate, with the same general principles, in organisms as different as yeast and man. The system is critical for a variety of physiological processes in which vesicle fusion must be controlled, ranging from signalling in the brain to release of hormones and immune cytokines. Defective vesicle transport occurs in a variety of diseases including a number of neurological and immunological disorders, as well as in diabetes. Without this wonderfully precise organization, the cell would lapse into chaos.
James E. Rothman was born 1950 in Haverhill, Massachusetts, USA. He received his PhD from Harvard Medical School in 1976, was a postdoctoral fellow at Massachusetts Institute of Technology, and moved in 1978 to Stanford University in California, where he started his research on the vesicles of the cell. Rothman has also worked at Princeton University, Memorial Sloan-Kettering Cancer Institute and Columbia University. In 2008, he joined the faculty of Yale University in New Haven, Connecticut, USA, where he is currently Professor and Chairman in the Department of Cell Biology.
Randy W. Schekman was born 1948 in St Paul, Minnesota, USA, studied at the University of California in Los Angeles and at Stanford University, where he obtained his PhD in 1974 under the supervision of Arthur Kornberg (Nobel Prize 1959) and in the same department that Rothman joined a few years later. In 1976, Schekman joined the faculty of the University of California at Berkeley, where he is currently Professor in the Department of Molecular and Cell biology. Schekman is also an investigator of Howard Hughes Medical Institute.
Thomas C. Südhof was born in 1955 in Göttingen, Germany. He studied at the Georg-August-Universität in Göttingen, where he received an MD in 1982 and a Doctorate in neurochemistry the same year. In 1983, he moved to the University of Texas Southwestern Medical Center in Dallas, Texas, USA, as a postdoctoral fellow with Michael Brown and Joseph Goldstein (who shared the 1985 Nobel Prize in Physiology or Medicine). Südhof became an investigator of Howard Hughes Medical Institute in 1991 and was appointed Professor of Molecular and Cellular Physiology at Stanford University in 2008.
Miniature human liver grown in mice. Transplanting tiny 'liver buds' constructed from human stem cells restores liver function in mice, researchers have found. Although preliminary, the results offer a potential path towards developing treatments for the thousands of patients awaiting liver transplants every year. The liver buds, approximately 4 mm across, staved off death in mice with liver failure, the researchers report this week in Nature.
The transplanted structures also took on a range of liver functions — secreting liver-specific proteins and producing human-specific metabolites. But perhaps most notably, these buds quickly hooked up with nearby blood vessels and continued to grow after transplantation. The results are preliminary but promising, says Valerie Gouon-Evans, who studies liver development and regeneration at Mount Sinai Hospital in New York. “This is a very novel thing,” she says. Because the liver buds are supported by the host’s blood system, transplanted cells can continue to proliferate and perform liver functions.
However, she says, the transplanted animals need to be observed for several more months to see whether the cells begin to degenerate or form tumours.
There is a dire scarcity of human livers for transplant. In 2011, 5,805 adult liver transplants were done in the United States. That same year, 2,938 people died waiting for new livers or became too sick to remain on waiting lists.
However, attempts to create complex organs in the laboratory have been challenging. Takanori Takebe, a stem-cell biologist at Yokohama City University in Japan who co-led the study, believes this is the first time that people have made a solid organ using induced pluripotent stem cells, which are created by reprogramming mature skin cells to an embryo-like state.
Testing whether liver buds could help sick patients is years away, says Takebe. Apart from the need for longer-term experiments in animals, it is not yet possible to make liver buds in quantities sufficient for human transplantation.
In the current work, Takebe transplanted buds surgically at sites in the cranium or the abdomen. In future work, Takebe hopes to create liver buds small enough to be delivered intravenously in mice and, eventually, in humans. He also hopes to transplant the buds to the liver itself, where he hopes they will form bile ducts, which are important for proper digestion and were not observed in the latest study.
Self-organizing structures
The researchers make the liver buds from three types of human cells. First, they coax induced pluripotent stem cells into a cell type that expresses liver genes. Then they add endothelial cells (which line blood vessels) from umbilical cord blood, and mesenchymal stem cells, which can make bone, cartilage and fat. These cell types also come together as the liver begins to form in the developing embryo.
“It’s a great day for developmental biology,” says Kenneth Zaret, who studies regenerative medicine and liver development at the University of Pennsylvania in Philadelphia. “By reconstituting cell interactions that we know are important for natural liver progression, they get what appears to be robust, mature tissue.”
The project began with an unexpected phenomenon, says Takebe. Hoping to find ways of to make vascularized liver tissues, he tried culturing multiple cell types together and noticed that they began to self-organize into three-dimensional structures. From there, the process for making liver buds took hundreds of trials to tweak parameters such as the maturity and ratios of cells.
Other organs
This strategy takes a middle path between two common strategies in regenerative medicine. For simple, hollow organs such as the bladder and trachea, researchers seed scaffolds with living cells and then transplant the entire organ into patients. Researchers have also worked to create pure cultures of functional cells in the laboratory, hoping that cells could be infused into patients, where they would establish themselves. But even if the cells work perfectly in the laboratory, says Gouon-Evans, the process of harvesting cells can damage them and destroy their function.
Zaret thinks that the liver buds work might encourage an intermediate approach. “Basically, put the cells in a room together and let them talk to each other and make the organ.”
Self-organizing structures from stem cells have also been observed for other organ systems, such as the optic cup, an early structure in eye development2. And 'mini-guts' have been grown in culture from single human stem cells3.
Takebe believes that the self-organizing approach might also be applicable to other organs, such as lung, pancreas and kidney.
Regenerative Medicine Day at Skolkovo. February 14, 2013 declared as a “Regenerative medicine day in Russia” by Skolkovo Innovative Centre. The world well known scientists of the field made presentations in the Hypercube of Skolkovo.
Conference devoted to a cross-disciplinary (biology, medicine, physics, chemistry) science - regenerative medicine – was sponsored by the Russian company 3D bioprinting solutions (executive director Aleksandr Ostrovsky, chairman of the board of company grouping INVITRO).
A group of company’s scientists is already working at organs and tissues 3D printing technology from human autologous cells under the guidance of Vladimir Mironov - organ bioprinting technology pioneer and professor of Virginia Commonwealth University, USA. Since the autologous cells are isolated from patient’s body, this technology may become a solution to a problem of biocompatibility and opens up the possibility to create inexpensive organ prototypes and efficient ways of tissue substitution in the longer term.
Vladimir Mironov had a presentation at the Skolkovo conference, where he explained what the bioprinting is, told about pending issues of this technology and already implemented projects, among which are: a device for tissue spheroid creation and a patented technology for nanofiber vascular implant creation. Mironov said at the press-conference, that first simple organs will be printed by 2030.
3D-bioprinting – is a comparatively new and fast emerging sphere of regenerative medicine. The main point of the technology is that 3D-printer is filled in with cells, layer of which is put onto special surface and then it builds 3D-organ layer by layer. 3D-bioprintng is similar to 3D-printing technology, which allows creating almost everything by means of special printers. According to Helena Livshits, director of medical cluster Biomed of the Skolkovo Foundation, a motorcycle able to run is printed during a couple of days. 3D-bioprinting task is creation of human “spare parts”, says Aleksandr Ostrovsky, Invitro Company Group. “Medicine is getting more expensive, and if there is no way out, the bills may grow up to vast sums”, says he.
Bioprinting is an impressive direction of regenerative medicine, but not the only. Time shows, which technology is more effective, most likely it is going to be either a combination of various approaches or different solutions for certain organs and tissue types. The examples of these approaches were presented by the Professor of regenerative surgery of Karolinska Institute (Sweden), the winner of Megagrant of the Russian Federation in 2011, Paolo Macchiarini. In particular, he developed a technology for trachea creation for transplantation and performed the world first laryngo-tracheal transplantation with the use of patient’s stem cells in the frames of the Megagrant.
Among other contributors was professor Xuejun Wen, of Virginia Commonwealth University. He told about the computerized tissue creation for regenerative medicine.
The main topics of the panel discussions were the present stage of regenerative medicine in the world and in Russia, its development prospects, main participants of the process and the main trends. Gennadiy Konstantinov, professor of the Higher School of Economics, spoke to the problem of private equity in this science-based sphere. His presentation was followed by the panel discussion with the participation of the Foundation for Assistance to Small Innovative Enterprises in Science and Technology representatives (Ivan Bortnik’s Foundation), Russian venture company representatives, the founder of “Science for Life Extension” Foundation Mikhail Batin and other experts.
3-5 December, 2012 the annual World Stem Cell Summit, the global stem cell and regenerative medicine community’s flagship event was held. It is the largest interdisciplinary, networking meeting of stem cell stakeholders, uniting the diverse regenerative medicine community. With the overarching purpose of fostering biomedical research, funding and investments targeting cures, the Summit is the single conference charting the future of this burgeoning field.
Summit speakers have been featured on the national stage over the past few weeks. Paolo Macchiarini of the Karolinska Institute (who held plenary lecture “Stem-Cell Based Organ Replacement and Cell Therapy”), was featured in the New York Times for completing the first “bioartificial” organ transplant of its kind. Susan Solomon of the New York Stem Cell Foundation has received more than 17,000 hits for her TED Talk, "Realizing the Promise of Stem Cell Research," and George Daley of Children's Hospital Boston and Harvard University was interviewed on NPR for a story entitled, "Scientists Create Fertile Eggs From Mouse Stem Cells". Read more about some of our featured speakers here.
The program of the Summit provided the research, industry, economic and societal context for understanding how all of the pieces of the stem cell puzzle fit together. More than 150 speakers participated in the conference and 50 hours of in-depth presentations were done.
2012 Disease focuses included: Caner, Diabetes; HIV / AIDS; Cardiovascular; Spinal Cord Injury, Paralysis, Parkinson's and other Neurological Diseases; Diseases of the Eye and a few others.
The 2012 World Stem Cell Summit interdisciplinary program is designed to explore the field’s most pressing topics with four tracks:
-
1) Basic Research
-
2) Translation and Regulation
-
3) Commercialization and Reimbursements
-
4) Advocacy/Consumer Safety.
In addition to compelling keynotes and panel discussions, the diverse three-day program included Expert Lunch roundtables; an Exhibit Hall; the Poster Forum showcasing science and policy research; the gala Stem Cell Action Awards Dinner; as well as many networking and partnering opportunities.
Poster Forum candidates included scientists and researchers; representatives of industry; stem cell research funding organizations and economic development officials; members of Institutional Review Boards and Stem Cell Research Oversight Committees; patient advocacy organizations, patients, and representatives of related not-for-profit organizations; government agencies related to public health and their counterparts from around the globe; representatives from the insurance and finance industries; professors and students of medicine, physiology, biology, other relevant sciences, ethics, law, and other disciplines related to social implications; and practicing professionals. The winners of the World Stem Cell Summit Poster Prize were announced by Alan Jakimo, Senior Counsel, Sidley Austin.
Three winners were selected in the category of Science, Technology, and Industry; one winner in the category of Advocacy, Education, and Public Engagement; and one winner for the Student Prize for the most creative or innovative poster coming from a high school or undergraduate college student.
Winners will have the opportunity to publish their research in Regenerative Medicine journal. The first place overall winner will receive a choice of free registration to the 2013 World Stem Cell Summit or $500; second and third place winners will receive $250; fourth and fifth place winners will receive $100.
After the prizes were announced, Jakimo said, "Posters at conferences in any field are like new therapies in healthcare - a sign of hope. Based on the posters at this year's Summit, we have much about which to be hopeful."
John B. Gurdon of the Gurdon Institute in Cambridge and Shinya Yamanaka of Kyoto University in Japan have won the 2012 Nobel Prize for Physiology or Medicine for finding that cells of an adult organism—once thought be terminally locked into their developed state—can start anew. The discoveries, awarded the prize by The Nobel Assembly at Karolinska Institute in Stockholm, have ignited research in areas ranging from cloning to cancer treatment.
“Gurdon and Yamanaka fundamentally changed the way we all think about the specialized state of cells,” George Daley, director of the Stem Cell Transplantation Program at the Harvard Medical School. “Collectively they taught us that the identity of a cell can be re-engineered—that an adult cell can be reverted to its embryonic state. This paradigm-shifting concept has opened up whole new avenues of research.”
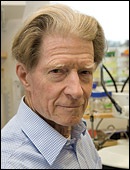 Gurdon, sometimes referred to as the “the godfather of cloning,” published a landmark study in the Journal of Embryology and Experimental Morphology in 1962, showing that implanting the nucleus of an adult frog cell into a frog egg that had had its nucleus removed could result in a functional, cloned tadpole. Though widely scrutinized at the time, Gurdon’s reprogrammed frog paved the way for breakthroughs in cloning, eventually leading to the creation of the now famous sheep, Dolly, in 1996.
Gurdon’s first nuclear-transfer experiment raised the possibility that adult cells maintain the ability to orchestrate embryonic development, despite having already reached their developmental fate. But a question remained about whether intact cells could be reprogrammed to a pluripotent state, capable of giving rise to other cell types of the body.
The answer came more than 40 years after Gurdon’s frog experiment, when Shinya Yamanaka discovered that four genes are capable of reprogramming an adult mouse cell to a cell with embryonic-like flexibility. In the first few days of development, pluripotent embryonic stem cells give rise to all the cells of the body, including those of the skin, intestines, and brain. Yamanaka’s finding showed that intact, adult cells can be rebooted into what are now known as induced pluripotent (iPS) cells, which, like embryonic stem cells, can give rise to many different cell types. The 2006 finding, published in Cell, provides researchers with the genetic recipe to generate iPS cells from mature cells in mice and humans.
Since the discovery, other scientists have generated iPS cells from humans. These iPS cells allow researchers to study disease and development in the lab by, for example, generating disease models from the skin of patients with neurodegenerative disorders. The cells are also showing promise for cell replacement therapies to treat various illnesses.
“Induced pluripotent stem cells have already begun to revolutionize medicine,” Paul Fairchild, director of the Oxford Stem Cell Institute. They provide “much-needed models of rare and complex disease states while providing sources of cells that may one day be used to replace those that are either worn out or compromised by degenerative diseases.”
The award is a long-time coming, said Magdalena Zernicka-Goetz, of the The Gurdon Institute of Cambridge. “I think we were all expecting it for quite a while,” she said.
3-rd Tissue Engineering and Regenerative Medicine Congress (5-8 September 2012, Vienna, Austria) brought together more than 3000 scientists all over the world represented different fields. Scientific program included about 300 oral presentations and 1500 posters.
The program reflects the rapid progress being made in this field during last 3 years, since the previous TERMIS congress.
This year the record number of presentations described the clinical translation of Tissue Engineering and Regenerative Medicine. You could find sessions focused on enabling technologies, cell therapy, scaffold based therapy, gene therapy, biomaterials, and these sessions covered all organ systems including the musculoskeletal system, central nervous system, cardiovascular, gastrointestinal, and oromaxillo-facial tissues. Membership in TERMIS has experienced robust growth (more than 4000 by now) with equal participation from the America’s, European, and Asia Pacific chapters. Each chapter has strong representation at the Congress.
The program also reflects the multidisciplinary peculiarity of regenerative medicine and at the same time - connection between basic and applied sciences. Several sections covered the basic issues of molecular and cell biology. Such as - Mechanism on Stem Cell Action (in coop. REMEDIC), keynotes: Paolo Bianco (Sapienza University of Rome, Italy), Frank Luyten (Division of Skeletal Tissue Engineering, KULeuven, Belgium), Katarina Le Blanc (Karolinska Institute, Sweden). REMEDIC is a Research Networking Program funded by the European Science Foundation. One of the central aims is to facilitate the exchange of ideas across disciplines in the regenerative medicine area. During recent years there has been considerable controversy as to the mechanism of action of cell therapies employing pluripotent adult stem cells. This symposium brought together leading experts in the field of regenerative medicine to discuss the latest developments in this current hot topic in stem cell biology.
Mesenchymal & Stem Cell Therapy: Current Status and Future Developments: intensive research in academic institutions and small and medium sized enterprises tries to bridge the gap from bench to bedside by using mesenychmal cells for the treatment of degenerative conditions or trauma. The International Society for Cellular Therapy, which fosters applied research in this field, and TERMIS have joined forces to present an overview on the latest developments in the generation and application of mesenychmal cells for tissue repair and immunomodulation.
Two keynote lectures focused on: the challenges and ways to optimize culture conditions for mesenchymal cells including transdifferentiation; and the safety aspects of clinical application of chondrocytes including genetic studies. Spotlight presentations highlighted recent developments to address neurological trauma via cellular therapy, to apply mesenchymal cells for immunomodulation and how to tackle the issue of controlled cell delivery.
The concept of tissue engineering for regenerative medicine usually requires a multicomponent strategy including the application of biomaterials and cells. It has been shown that matrix mimicking the in vivo environment of the target tissue, including composition, organization, texture and mechanical properties is crucial for the behavior of the cells, to migrate, survive and take up their natural functions in the specific tissue. Cells and matrix originating for natural sources in particular human placenta and amnion should hence be able to fulfill these criteria and are promising candidates to stimulate the regeneration of tissues. Session Extracellular Matrix and Cells from Natural Tissues evaluated the suitability of these biomaterials processed from natural extracellular matrix and primary human cells including stem cells to promote processes of wound healing and tissue regeneration.
The key tool of regenerative medicine: biomaterials, scaffolds and bioreactors’ technology were presented at the congress very well.
Biomaterials are key elements in regenerative medicine, beside cells and signalling molecules: they allow or inhibit the attachmentor integration of specific cells, they can deliver drugs and mediators or can guide regeneration processes within the patients’ body. For these purposes new and functionalized materials with specific properties are under development, e.g. materials offering niches for stem cells and influencing their differentiation or materials delivering mediators on a specific signal from the surrounding tissues or only in a defined cell type. In some areas, e.g. the treatment of burned wounds, the former therapy with cultivated cells is today often replaced by a treatment with highly specific biomaterials giving even better results. The symposium Biomaterials for Regenerative Medicine focused on some characteristics that could be achieved by designing appropriate biomaterials and on methods to prove functionality and safety.
Session 3D Scaffold/Cell Printing covered technological aspects regarding fabrication of 3D-scaffolds as well as the development of suitable biomaterials for scaffold fabrication. A special focus was on additive manufacturing techniques and techniques for cell culture in 3D. New methods, which allow the processing of biomaterials in the presence of living cells also were presented. Such as Laser fabrication of multi-scale elastic 3D scaffolds (Ovsianikov A, Dado-Rosenfeld D, Nurnberger S, Levenberg S, Redl H, Liska R, Stampfl J, Inst. of Materials Science and Technology TU Wien, Austria) or Self-assembly-based biofabrication (Marga F, Jakab K, Norotte C, Khatiwala C, Shepard B, Dorfman S, Colbert S, Hubbard B, Forgacs G, University of Missouri, USA).
The examples of the application and development of these techniques were presented by groups from Wake Forest Institute for Regenerative Medicine, USA (Development and validation of an integrated organ printing system, Kang HW, Kengla C, Lee SJ, Atala A, Yoo J) and Imperial College London, UK (New materials-based strategies for regenerative medicine, Stevens MM).
Bioreactor cultivation of three-dimensional tissue constructs allows controlled culture conditions to support tissue growth, to maintain tissue vitality and to induce and sustain tissue differentiation. In order to achieve reproducible culture conditions and results the cultivation process needs to be continuously supervised. Control units and sensor systems enable monitoring of tissue growth and provide data for establishing reproducible culture protocols and procedures. The challenges of automatization of these processes lie in the transfer of biological reactions with high variability into standardized and harmonized procedures for high-throughput tissue generation. Automatization of Bioreactor Technologies for 3D Tissue Cultures session covered the problems and challenges arising when manually-controlled individualized culture processes are transferred into reproducible and automatized cultivation procedures for Tissue Engineering.
Symposium Bioreactors for Translational Research focused on studies that involve the design and utilization of bioreactors for cultivation of human and animal cells and engineering of functional tissues for translational research. The areas of interest include (but are not limited):
-
engineering of tissue constructs for implantation, using stem, progenitor, differentiated cells, alone or in conjunction with biomaterial scaffolds,
-
studies of disease using bioreactors with cell aggregates or engineered tissues,
-
studies of stem cells, including directed differentiation, expansion and characterization of differentiation capacity.
The session included high-quality presentations from different areas of basic and applied research and technology development that can advance and facilitate translation of tissue engineering research into clinical applications.
The translational researches, as mentioned already, and trends of clinical applications in the area was the most important and representative part of the congress. Every case of translational research in regenerative medicine covers clinical translation of cell therapy, tissue, organ regeneration and therapeutic biomaterials development. You could see the examples of different approaches performed on the different types of tissues and organs.
Trachea/Oesophagus/Chest – Repair and Replacement. Both malignant and benign disorders affecting the thorax can be surgically rejected but majority have an inoperable size at the time of diagnosis. Due to a lack of reconstructive tissue and optimal solutions, new therapeutic options are needed. Recently, tissue engineering and regenerative approaches demonstrated their great potential in both experimental and clinical conditions with respect to several different complex tissues, such as the trachea, urinary bladder, blood vessels, cartilage, skin and heart valves. The discovery of novel mechanisms and technologies will get us one step closer to routine clinical application. Symposia keynote Philipp Jungebluth (Karolinska Institute, Sweden) made an analytical presentation based on the practical things – examples of successful clinical trachea transplantations, made by Professor Paolo Macchiarini and his group. (Tissue engineering – from bench to bedside and back to bench).
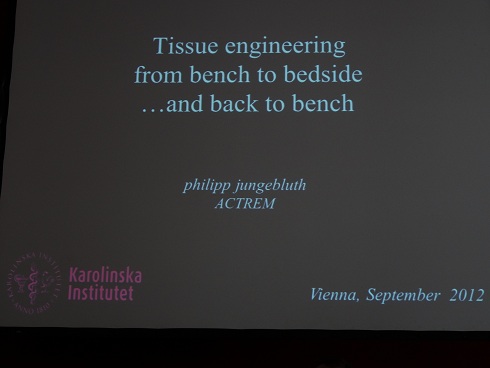 Key sections on other organs and tissues: Preformed Vasculature and Induced Angiogenesis, Heart, Pancreas, Liver, Bone, Skin Regeneration, Spinal Cord Injury.
Such a complicated field requires a lot of analytic work, which were presented in the plenary lectures. The authors tried to show the trends of regenerative medicine’ development.
The speakers were:
Dietmar W. Hutmacher - Professor and Chair of Regenerative Medicine, Institute of Health and Biomedical Innovation of QUT. He leads the Regenerative Medicine Group, a multidisciplinary team of researchers including engineers, cell biologists, polymer chemists, clinicians, and veterinary surgeons. His team is endeavoring to meet the challenge to provide new bone to replace or restore the function of traumatized bone or bone lost as a consequence of age or disease. Bone tissue engineering concepts developed by his group promise to deliver specifiable replacement tissues and the prospect of efficacious alternative therapies for orthopedic applications such as non-union fractures, healing of critical-sized segmental defects and regeneration. Another key project: Professor Hutmacher’s group has been developed an animal model for bone repair research. Finally, the group is using their expertise in biomaterials & tissue engineering to tackle a different problem: development of 3D cancer models in the lab from scratch.
(Plenary Debate: Future of publishing)
David Kaplan - Professor & Chair of the Department of Biomedical Engineering and also holds faculty appointments in the School of Medicine, the School of Dental Medicine, Department of Chemistry and the Department of Chemical and Biological Engineering (Tufts University). His research focus is on biopolymer engineering to understand structure-function relationships, with emphasis on studies related to self-assembly, biomaterials engineering and functional tissue engineering. He directs the NIH P41 Tissue Engineering Resource Centre (TERC) that involves Tufts University and Columbia University.
(Lecture: Human disease models through tissue engineering)
Ivan Martin – Director of the Tissue Engineering Research Group, Professor for Tissue Engineering, Faculty of Medicine Departments of Surgery and of Biomedicine, University of Basel. His group includes scientists from the biological, engineering and clinical fields, allowing interdisciplinary work with a strong translational component. His research interests are centered on 3D culture systems for mesenchymal cells of various origins, as models to study cell differentiation and tissue development, or as grafts for bone and cartilage repair.
(Lecture: Paradigm Shift: Evolution of tissue engineering Strategies)
Shinichi Nishikawa - Director of the laboratory for Stem Cell Biology at RIKEN Centre for Developmental Biology (CDB) and also Deputy Director of CDB, Japan. The major interest of his group is the molecular and cellular mechanisms governing development of hematopoietic stem cells. While his research interest is diverse, he made important contributions to each field through t works on the differentiation of B-cells, the function of tyrosine kinase receptors of the PDGFR family, the differentiation of hematopoietic stem cells and the development of peripheral lymphatic tissues. Professor Nishikawa became one of the leading stem cell biologists.
(Lecture: Overcoming problems of iPS based cell therapy: Japanese national project for realization of Regenerative Medicine)
Clemens A. van Blitterswijk - Professor of Tissue Regeneration at Twente University in the Netherlands, most of his research deals with Tissue Engineering and Regenerative Medicine forming a unique basis of multidisciplinary research between the materials and life sciences. His group got the maximum score of 4 fives in the QANU Research Review Chemical Engineering 3TU (evaluation of chemical engineering), 2010. Now he heads one of the leading European labs in the field of Tissue Engineering and Regenerative Medicine, he combines this task with the scientific directorship of MIRA, a recently established institute for biomedical technology and technical medicine, which will grow to over 400 staff in the coming years.
(Lecture: Paradigm Shift: A high throughput toolbox for tissue engineering)
David Williams - Editor-in-Chief of Biomaterials. He has had 40 years’ experience in the biomaterials, medical device and tissue engineering fields. Professor Williams left the University of Liverpool, UK, in 2007 from the position of Head of Clinical Engineering.
He is currently Professor and Director of International Affairs, Wake Forest Institute of Regenerative Medicine, North Carolina, USA, and a Visiting Professor in Cape Town, Sydney, Beijing, Shanghai and Taipei. He is President Elect of the Tissue Engineering & Regenerative Medicine International Society.
(Plenary Debate: Future of publishing)
The regenerative medicine as a new field (TERMIS founded about 10 years ago) requires the regulation. We have not the unified rules in different countries so far, that is why the organizers paid attention to this subject inviting the representatives of two most authoritative organizations - European Medicines Agency [EMEA] (D’Apote L.)and FDA‘s Center for Biologics Evaluation and Research [CBER], USA (Witten C). They presented lectures on the regulatory environment of regenerative medicine in USA and Europe. The key point of both presentations: as science (and translational research as well) becomes more international, we need more cooperation and integration in order to unify the rules.
The organizers also paid special attention to the students and young scientists and tried to give them an opportunity to learn. There were several special events, like “Meet Mentor”, where the students had a chance to talk and discuss their field of interest and even careers with the distinguish scientists. They also had the opportunity to assist chairing a session with a leader in the research field.
The next TERMIS World Congress will take place in September 2015 in Boston (USA).
The 3rd World Congress TERMIS «Tissue Engineering and Regenerative Medicine» will take place in Vienna in September 5-8, 2012. The TERMIS is the premier location for the international community of TERMIS members to present their research and interact with top researchers, clinicians, scientists, students and industry.
The major goals of the meeting are:
- to discuss the translational aspects of tissue engineering and regenerative medicine
- to promote young researchers
- to connect with other societies concerning important aspects of tissue regeneration
- to provide a platform for fruitful exchange between industry and academics
http://www.wc2012-vienna.org
A òew Center of Regenerative Medicine, one of the biggest in Europe, was open in Edinburgh. The £54million building is the first large-scale, purpose-built facility of its kind in the UK.
A NEW research centre to find treatments for major diseases has been opened today by the Princess Royal. The Scottish Centre for Regenerative Medicine in Edinburgh will house scientists looking at conditions such as multiple sclerosis and heart and liver disease. They will carry out cutting-edge stem cell research to help find therapies for patients with conditions including Parkinson's disease and motor neurone disease.
The £54million building is the first large-scale, purpose-built facility of its kind in the UK. It is funded by the University of Edinburgh, Scottish Enterprise, the Medical Research Council (MRC) and the British Heart Foundation through its Mending Broken Hearts Appeal.
It includes the most up-to-date facilities in the UK to manufacture stem cell lines that could be used for patient therapies. Based in Edinburgh's BioQuarter, it was built in a partnership between Scottish Enterprise, the University of Edinburgh, NHS Lothian and Alexandria Real Estate Equities. It is expected to become the first laboratory in Scotland to be given a building research establishment environmental assessment method (BREEAM) excellent rating for its sustainability, choice of materials and the efficiency of its design and details.
Full Text
http://www.dailyrecord.co.uk/news/scottish-news/2012/05/28/princess-ann-opens-new-research-centre-for-major-diseases-in-edinburgh-86908-23877122/
A team, led by Prof Alexander Seifalian of the Department of Nanotechnology and Regenerative Medicine in University College, London, claims it's actually focusing on growing replacement organs and body parts to order, using a patient's own cells.
6 May 2012
'This is a nose we’re growing for a patient next month,’ Professor Alexander Seifalian says matter-of-factly, plucking a Petri dish from the bench beside him.
Inside is an utterly lifelike appendage, swimming in red goo. Alongside it is another dish containing an ear.
‘It’s a world first,’ he says smiling.
‘Nobody has ever grown a nose before.’
His lab is little more than a series of worn wooden desktops strewn with beakers, solutions, taps, medical jars, tubing and paperwork, and looks like a school chemistry lab.
But it’s from here that Seifalian leads University College London’s (UCL) Department of Nanotechnology and Regenerative Medicine, which he jokingly calls the ‘human body parts store’.
As he takes me on a tour of his lab I’m bombarded with one medical breakthrough after another.
At one desk he picks up a glass mould that shaped the trachea – windpipe – used in the world’s first synthetic organ transplant.
At another are the ingredients for the revolutionary nanomaterial at the heart of his creations, and just beyond that is a large machine with a pale, gossamer-thin cable inside that’s pulsing with what looks like a heartbeat. It’s an artery.
Full text
Regenerative medicine repairs mice from top to toe. Three studies published in April 2012 in Nature magazines show that introducing new cells into mice can replace diseased cells — whether hair, eye or heart — and help to restore the normal function of those cells. These proof-of-principle studies now have researchers setting their sights on clinical trials to see if the procedures could work in humans.
18 April 2012
At the turn of the twentieth century, the promise of regenerating damaged tissue was so far-fetched that Thomas Hunt Morgan, despairing that his work on earthworms could ever be applied to humans, abandoned the field to study heredity instead. Though he won the Nobel Prize in 1933 for his work on the role of chromosomes in inheritance, if he lived today, the advances in regenerative medicine may have tempted him to reconsider.
Three studies published this week show that introducing new cells into mice can replace diseased cells — whether hair, eye or heart — and help to restore the normal function of those cells. These proof-of-principle studies now have researchers setting their sights on clinical trials to see if the procedures could work in humans.
“You can grow cells in a Petri dish, but that’s not regenerative medicine,” says Robin Ali, a geneticist at University College London, who led the eye study. “You have to think about the biology of repair in a living system.”
http://www.nature.com/news/regenerative-medicine-repairs-mice-from-top-to-toe-1.10472
Engineering whole organs: closing in on a potential solution to the organ donor shortage?
A new technique involving the use of an artificial scaffold into which a patient’s own stem cells are inserted, turning it into a fully functional organ, could offer a potential solution to the donor shortage crisis, according to the second paper in this week’s Lancet Series on stem cells. This pioneering approach to regenerating and transplanting organs requires no human donors, has no problems with rejection, and has no need for immunosuppressive drugs.
“Such an approach has already been used successfully for the repair and reconstruction of several complex tissues such as the trachea, oesophagus, and skeletal muscle in animal models and human beings, and guided by appropriate scientific and ethical oversight, could serve as a platform for the engineering of whole organs and other tissues, and might become a viable and practical future therapeutic approach to meet demand after organ failure”, explains Paolo Macchiarini from the Karolinska Institutet, Stockholm, Sweden, lead author of the paper.
Because of an ageing population there is a growing crisis in whole-organ donor supply. Every year in the USA alone, about 120 000 people die from chronic lung disease, 112 000 from kidney failure, and 425 000 from coronary heart disease. Patients who are fortunate enough to receive a donor organ still face life-long expensive and potentially dangerous immunosuppressive therapy.
In this paper, Macchiarini and colleagues discuss the use of a new regenerative technique based on the use of naturally occurring extracellular matrix as a biological scaffold, outline the key scientific and ethical challenges that remain before wider clinical use of this approach is possible, and review progress made in the reconstruction of individual organs.
Optimum cell source for different organs, ideal scaffold material, and identification of the appropriate population of patients are some of the key questions that will need to be addressed before widespread clinical use.
“For clinical trials, due consideration needs to be given to who to recruit: suitable patients should be able to provide competent consent, have some amount of social support, have few comorbidities, and be willing to face loss of privacy”, say the authors.
As well as scientific challenges, more needs to be done to address the numerous ethical issues raised by this new technology. “The pressure to advance this technique, driven by demand, the race for prestige, and the potential for huge profits, mandates an early commitment be made to establish the safety of various strategies…particularly when there are so many potential patients and doctors who are desperate for any remedy that offers hope”, warn Macchiarini and colleagues.
They conclude by calling for policies to address issues including: transparency about the techniques involved, cell sources, financial costs to patients, informed consent, strategies for dealing with experimental failure, and assisting patients after initial treatment, adding that: “Perhaps the strongest ethical duty the bioengineering community faces is the identification of criteria that constitute sufficient evidence of the evolution of an intervention from research to therapy…Establishment of adequate safety and functional success will need input from investigators and key professional societies and organisations.”
In an accompanying Comment, Dusko Ilic from King’s College Medical School, London, and Julia Polak from Imperial College, London remark: “Although several questions are unresolved, the promise of an off-the-shelf scaffold that can be repopulated with autologous stem cells expanded in vitro seems much closer than one could have hoped for even a few years ago.”
Professor Paolo Macchiarini, Karolinska Institutet, Stockholm, Sweden.
paolo.macchiarini@ki.se
Dr Dusko Ilic, King’s College Medical School, London, UK.
dusko.ilic@kcl.ac.uk
Compaction enhances extracellular matrix content and mechanical properties of tissue engineered cartilaginous constructs
Scientiests from the University of California, San Diego, hypothesized that the load-bearing mechanical properties of cartilaginous constructs improve with the inclusion of collagen (COL) and proteoglycan (PG) during assembly. The objectives of this work were to determine the effect of addition of PG, COL, or COL+PG on compressive properties of 2% agarose constructs and the ability of mechanical compaction to concentrate matrix content and improve the compressive properties of such constructs. The inclusion of COL+PG improved the compressive properties of hydrogel constructs compared to PG or COL alone. These results support the concepts that the assembly of cartilaginous constructs with COL+PG and application of mechanical compaction enhance the ECM content and compressive properties of engineered cartilaginous constructs.
http://online.liebertpub.com/doi/abs/10.1089/ten.TEA.2011.0300?elq=7c3e9838d6b34dce8303e9490bbf0a55
Hydrogel derived from decellularized ventricular extracellular matrix increases cardiomyocyte survival and preserves post-myocardial infarction
The study reported in the Journal American College of Cardiology evaluated the use of an injectable hydrogel derived from ventricular extracellular matrix (ECM) for treating myocardial infarction (MI) and its ability to be delivered percutaneously. There was developed a myocardial-specific hydrogel, derived from decellularized ventricular ECM of female rats underwent ischemia reperfusion followed by injection of the hydrogel or saline 2 weeks later. The hydrogel was delivered by the NOGA-guided MyoStar catheter (California). There was demonstrated that injection of the material in the rat MI model increases endogenous cardiomyocytes in the infarct area and maintains cardiac function without inducing arrhythmias. The results warrant further study of this material in a large animal model of MI and suggest this may be a promising new therapy for treating MI.
http://content.onlinejacc.org/cgi/content/citation/57/14_Suppl_S/E2017?elq=92a7cb4ee9df4c99974e5cce5c89a9f6
Biomechanical and angiogenic properties of tissue-engineered rat trachea using genipin cross-linked decellularized tissue
As Biomaterials reports, in the study of italian scientists headed by Professor Paolo Macchiarini, the obtainment and characterization of decellularized rat tracheal grafts are described. The detergent-enzymatic method, already used to develop bioengineered pig and human trachea scaffolds, has been applied to rat tracheae in order to obtain airway grafts suitable to be used to improve our knowledge on the process of tissue-engineered airway transplantation and regeneration. The results demonstrated that, after 9 detergent-enzymatic cycles, almost complete decellularized tracheae, retaining mechanical properties of the native tissues with strong in vivo angiogenic characteristics, could be obtained. Moreover, to improve the mechanical properties of decellularized tracheae, genipin is here considered as a naturally derived cross-linking agent. The results demonstrated that the treatment increased mechanical properties, in term of secant modulus, without neither altering the pro-angiogenic properties of decellularized airway matrices or eliciting an in vivo inflammatory response.
http://www.sciencedirect.com/science/article/pii/S0142961211012117
The Use of Human Sweat Gland–Derived Stem Cells for Enhancing Vascularization during Dermal Regeneration
Stem cells derived from human sweat glands were isolated, characterized, seeded in collagen scaffolds, and engrafted in a mouse full skin defect model for dermal regeneration. Results - as Journal of Investigative Dermatology reports - showed that these cells exhibit high proliferation rates and express stem cell and differentiation markers. Moreover, cells responded to angiogenic environments by increasing their migration (P<0.001) and proliferation (P<0.05) capacity and forming capillary-like structures. After seeding in the scaffolds, cells distributed homogeneously, interacting directly with the scaffold, and released bioactive molecules involved in angiogenesis, immune response, and tissue remodeling. As autologous sweat gland–derived stem cells are easy to obtain, exhibit a good proliferation capacity, and improve vascularization during dermal regeneration, there were suggested to use them for dermal regeneration in future clinical settings.
http://www.nature.com/jid/journal/vaop/ncurrent/full/jid201231a.html?elq=b4a9aaedd1be498d978f99b7e0121931
Human cerebral cortex development from pluripotent stem cells to functional excitatory synapses
As Nature Neuroscience reports, there was developed a robust, multistep process for human cortical development from pluripotent stem cells: directed differentiation of human embryonic stem (ES) and induced pluripotent stem (iPS) cells to cortical stem and progenitor cells, followed by an extended period of cortical neurogenesis, neuronal terminal differentiation to acquire mature electrophysiological properties, and functional excitatory synaptic network formation. There was founded that induction of cortical neuroepithelial stem cells from human ES cells and iPS cell differentiation to cerebral cortex recapitulated in vivo development to generate all classes of cortical projection neurons in a fixed temporal order. This system enables functional studies of human cerebral cortex development and the generation of individual-specific cortical networks ex vivo for disease modeling and therapeutic purposes.
http://www.nature.com/neuro/journal/v15/n3/full/nn.3041.html
|